Research
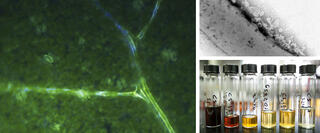
At Great Lakes Bioenergy, we draw on the expertise of engineers, economists, microbiologists, and ecologists to develop sustainable biofuels and bioproducts. Working together, we engineer bioenergy crops to enhance their environmental and economic value, conduct research to generate multiple products from plant biomass, and optimize the field-to-product pipeline.
Sustainable bioenergy cropping systems
Engineering bioenergy crops to enhance their environmental and economic value
Our researchers are improving the yield and processing traits of dedicated bioenergy crops for cultivation on marginal, nonagricultural lands. With smart management, these crops have the potential to benefit the ecosystem, help mitigate climate change, and provide farmers with an additional source of revenue.
Sustainable biomass conversion
Generating multiple products from plant biomass
Our researchers are finding new ways to process biomass at low cost, produce fuels for use in multiple engine types, and convert as much plant biomass as possible into biofuels and bioproducts. Our goal is to generate multiple products from biomass and enable a new generation of biorefineries.
Sustainable field-to-product optimization
Understanding and optimizing the field-to-product pipeline
GLBRC research is integrated across many disciplines and areas of focus. We understand our work as part of the field-to-product pipeline and coordinate our efforts to overcome the barriers to producing sustainable biofuels and bioproducts.