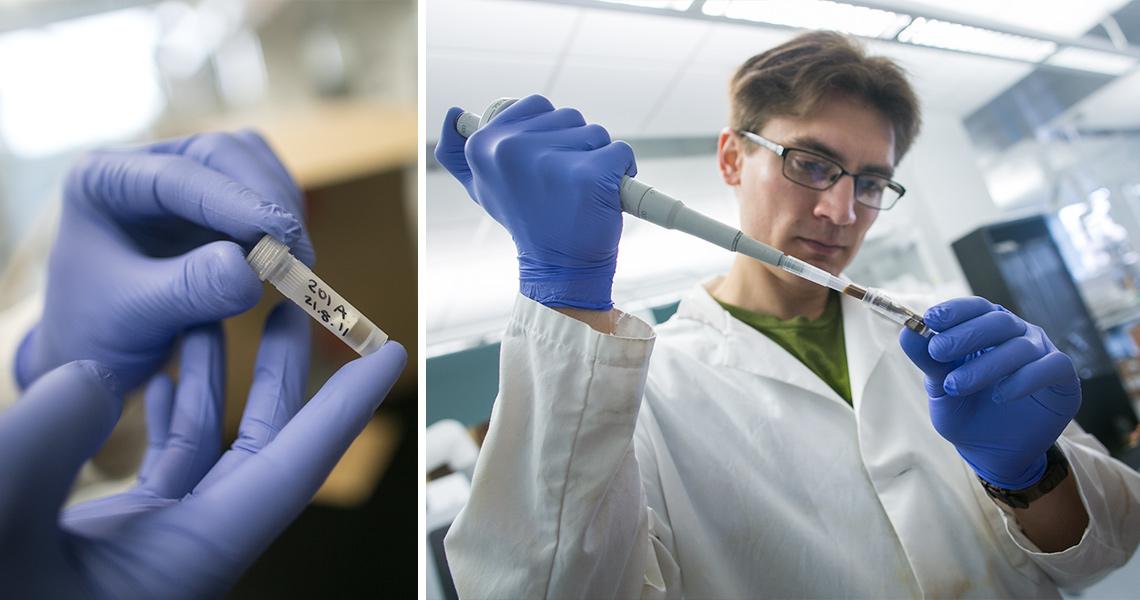
David Duncan loves to think about dirt, and a quick glance at his family tree could lead one to believe he comes by it naturally. His grandfather was an agricultural extension agent and his handful of uncles includes two agronomists and an expert on fungi.
But Duncan, a University of Wisconsin–Madison doctoral student in agronomy and a Great Lakes Bioenergy Research Center researcher, asserts that what really pulled him into agronomy was his mother.
“My interest in biology was agricultural from the get-go and I think it’s because my mom always loved the idea of having a great big garden,” Duncan says. “Early on, I learned from her that growing things was a good pursuit, something worth doing.”
Duncan spent his childhood in central Wyoming, an arid region where it’s hard to grow much of anything. He recalls tending some strawberries and rhubarb with his mom, but remembers even better the summers he spent digging around in the decomposing pile of grass clippings out by the shed.
“It was sort of an early experiment in microbial ecology,” Duncan says. “Unfortunately, it ended when the hornets set up a nest there.”
Boy scouts and soccer eventually displaced the compost pile, but Duncan ventured back to biology as an undergraduate at Stanford University where he studied plant biology and biotechnology and became interested in understanding the ecology of sustainable cropping systems.
Duncan graduated from Stanford in 2006 and joined UW–Madison’s College of Agricultural and Life Sciences master’s program in agroecology in 2007.
In 2010, he became a doctoral student in agronomy where his study of cropping systems brought him right back to the microbial ecology he enjoyed as a boy.
Today, when Duncan looks at dirt, he sees habitat for the millions of different species of microbes residing there.
“Microbes are incredibly charismatic if you have the right mindset,” says Duncan. “They’re quirky. They do things that don’t make sense at the human scale. For example, there are microbes that split up metabolic processes among multiple different organisms … kind of like you eating something and then your partner being the one to actually digest it.”
Microbes, the oldest life form on earth, are single-cell organisms so tiny that billions can live in a thimbleful of soil. But Duncan, whose research focuses on sustainable biofuel feedstocks, is searching agricultural fields for something even smaller – a single microbial gene called nitrous oxide reductase (nosZ) that’s responsible for making the loss of agricultural nitrogen less harmful to the environment.
When farmers apply nitrogen fertilizer to crops, the fertilizer is eventually transformed into nitrate. Nitrate is a simple form of nitrogen that’s easy for plants to use, but can also be lost in run-off or end up in the soil. Nitrogen loss is bad for farmers because they have to pay for the fertilizer, but even worse is what what nitrogen turns into when you lose it: nitrates in your drinking water and nitrous oxide in the atmosphere.
Nitrous oxide is a significant ozone-depleting chemical and a highly potent greenhouse gas, and agricultural use of fertilizers plays a significant role in its production. In fact, nitrogen loss from agricultural soil accounts for about 75% of total U.S. nitrous oxide emissions.
Duncan is comparing cropping systems including corn, switchgrass, and native prairie grass in an effort to understand how the microbial community as well as system variables such as crop type, temperature, moisture, and nitrogen availability contribute to nitrous oxide emissions. And the nosZ gene is a central focus of his research.
Some microbes have a group of genes that allows them to “breathe” nitrate. When microbes with an active nosZ gene breathe nitrate, it’s chemically converted to the harmless nitrogen gas that comprises 70% of our atmosphere. For microbes lacking an active nosZ gene, however, the nitrate conversion process produces nitrous oxide.
“If the nosZ gene does it job,” Duncan says, “we don’t see nitrous oxide emissions. But if nosZ is absent, or not working, then we see a lot of nitrous oxide emissions.”
Duncan would like to use the prevalence of nosZ to predict whether the nitrogen conversion process in a particular soil will be environmentally harmful. But it’s not that simple – sometimes, active microbes with the nosZ conversion ability don’t convert nitrogen at all.
For the nosZ conversion of nitrogen to take place, a number of conditions have to be met: (1) The microbe has to have the nosZ gene (many don’t); (2) The microbe has to be “awake” (many are inactive in a state resembling hibernation); and (3) The nosZ gene has to be “turned on” (in scientific terms, “expressed”).
Duncan’s work looks closely at both the microbial community and the environmental variables in order to understand the conditions that best foster the presence and wakefulness of nosZ microbes as well as the “turning on” of the nosZ gene.
“It’s important to have both research components in place,” Duncan explains, “because variables such as temperature, moisture, and nitrogen availability determine whether the microbes with the preferred conversion ability are awake and ready to render excess nitrogen harmless.”
While many of Duncan's research hours are spent in the field — sampling soils and documenting nitrous oxide emissions — far more of his time is spent analyzing vast digital datasets of microbial genetic data, looking at the abundance and types of nosZ present in agricultural soils.
Making sense of the data generated by sequencing microbial DNA is complicated, in part because most sequencing technologies have been developed to analyze human DNA. Any two people will have DNA sequences that are about 99.9% identical. In sharp contrast, just one gram of soil can contain somewhere between one and ten million different species of microbes, and some of these species are nothing alike.
Duncan, however, appears completely undaunted by the complexity.
“I’m happiest when I’m doing data analysis,” Duncan explains. “There's something extremely rewarding about uncovering patterns in your data, particularly when they’re patterns you can fit into a biologically coherent narrative.”
Duncan’s careful analysis of local soil is helping create a coherent narrative for biofuel cropping systems. His work is a starting point for understanding the complex relationships among energy crops, microbial communities, environmental variables, and nitrogen loss.
“Currently, we plant crops and measure nitrous oxide emissions, which is laborious,” Duncan says. “But we’re working to get to the point where we could instead use soil analysis, including nosZ content, to predict potential emissions.”
Short-term, Duncan believes his research can help both farmers and scientists evaluate potential bioenergy crops. Long-term, Duncan hopes his work will help scientists predict the environmental effects of different biofuel cropping systems in changing climates across the world.